Boxtahedral Tower
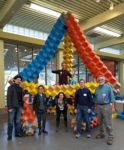
At last the day came for the installation of the Boxtahedral Tower at the Golden Gate Stem Fair. Below is our materials list and a picture of them waiting to be set up.
Read MoreAt last the day came for the installation of the Boxtahedral Tower at the Golden Gate Stem Fair. Below is our materials list and a picture of them waiting to be set up.
Read MoreIn addition to the main, planned build at the Golden Gate STEM Fair, and thanks to donations of materials from Primed Minds, there was also a do-it-yourself/take-home table at the STEM Fair. Participants produced such towers as this one:
Read MoreA brief post about the importance of prepping and prototyping for a build, and a couple of things that came up in the prep for the Golden Gate STEM Fair event. First, there’s just the sheer volume of supplies for such an event. Here are lots of beads getting outfitted with twist ties, for example.
Read MoreOk, all of the ingredients were in place to plan a large-scale construction for the Golden Gate STEM Fair: cubical units that can attach at edges and the theory linking them to the oct-tet lattice. I just needed to put it all together into a plan for something interesting and substantial that could be built out of lots of 6″ cubes (but not too many). Since height is a key factor in drawing attention, a nice round figure of 5 meters seemed like a good goal height for the structure. But what to build?
Read MoreAs a result of the last couple of constructions, when Studio Infinity signed up to do a large-scale construction at the Golden Gate STEM Fair, I had the oct-tet lattice on my mind. And for a long time, I had wanted to exploit the connection between cubes and the oct-tet lattice. I just needed a way to connect cubes edge-to-edge.
Read MoreSo when I was doing the math for the styrofoam-dowel tetrahedron, I noticed that locating the holes necessary for making a regular octahedron should be easy too. Naturally, having done the theory, it’s hard to resist actually building the thing.
Read MoreRecently a friend of mine was giving a (math) talk and wanted as a prop “a large tetrahedron with the vertices emphasized.” This seemed like a natural for Studio Infinity, so the assignment was accepted.
Read MoreWelcome to Studio Infinity! You most likely reached this page via the link on the Gengzhi Goblets given away at G4G13. Here are pointers to more in-depth discussions of the Goblets.
Read MoreSo far, we’ve created a lot of interesting small models of tensegrity structures. However, for doing public programs of the sort Storm King Art Center was planning, it’s always helpful to be able to build much larger models of things. Building giant models seems to get the ideas across more vividly, engage visitors more thoroughly, and be just plain fun.
Read MoreSo why the interest in tensegrity here at Studio Infinity? It begins with Kenneth Snelson, the inventor of tensegrity (although perhaps not of the term) as a student of Buckminster Fuller. Ken went on to become a noted sculptor, using tensegrity in many of his works. One of those sculptures, Free Ride Home, resides at Storm King Art Center. And recently, the education department at Storm King Art Center invited me to give a workshop there about tensegrity, how to make simple models of tensegrity, and how to share ideas about tensegrity with the public visiting the Center.
Read More